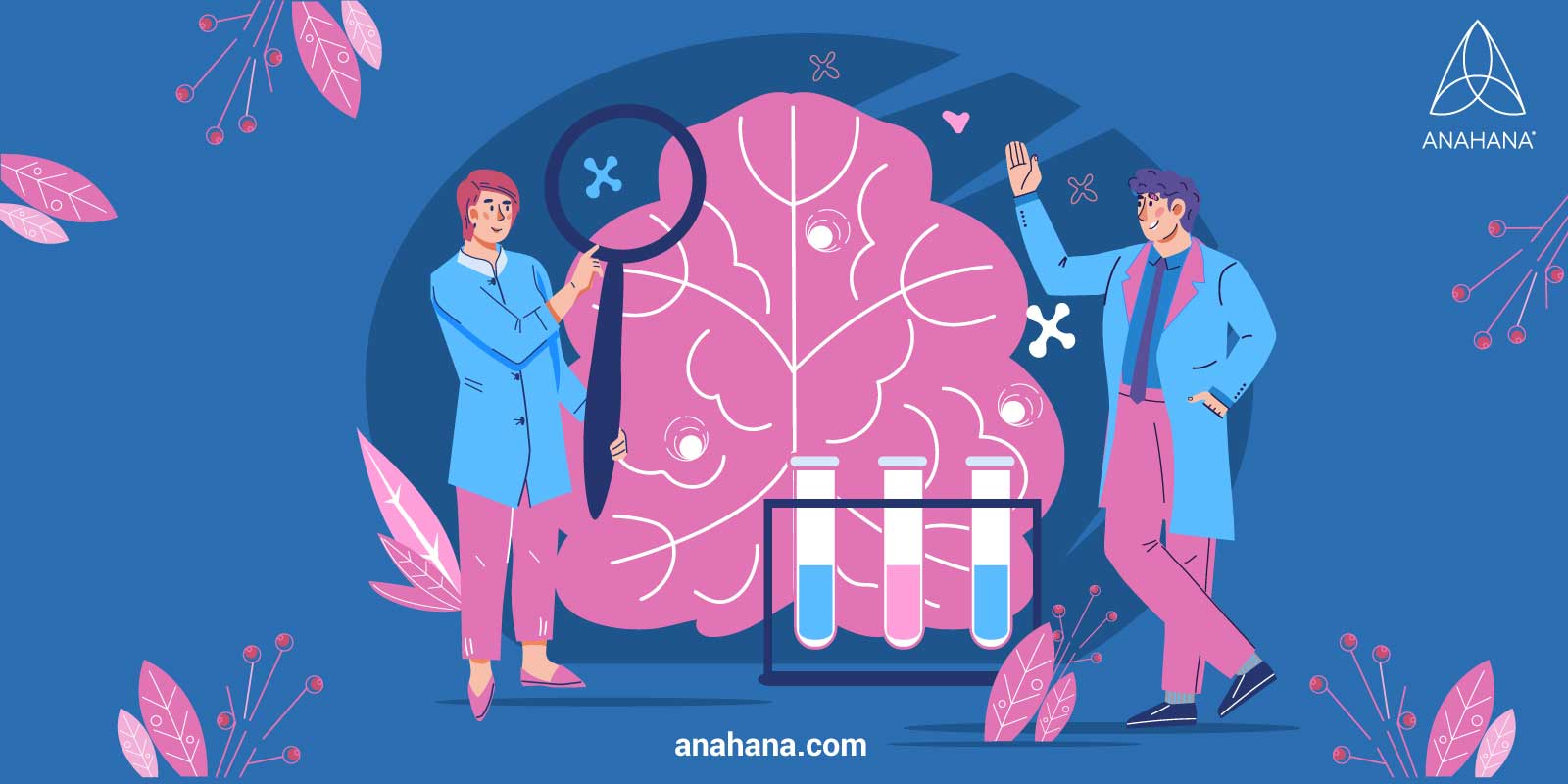
Table of Contents
Neuroplasticity describes the brain’s ability to change and adapt. The brain is a remarkably malleable organ. As we grow and learn, our experiences multiply, and our brain cells evolve. These structural alterations create neural pathways that allow us to apply what we learned in the past to new challenges.
The human brain can pull off some of the most astonishing recovery journeys. We hear stories of stroke patients re-learning to read and write and athletes re-gaining their fine motor skills following traumatic brain injuries. These feats are made possible by our nervous system’s powerful plasticity.
The central nervous system (the brain and spinal cord) is the root of all thought, movement, emotion, and memory - in essence, the human experience. To understand neuroplasticity is to comprehend the dynamic nature of our brain and the rest of the nervous system. From there, we may begin to glimpse how we can harness this potential.
What is Neuroplasticity?
Neuroplasticity is an umbrella term that describes the brain’s exceptional capacity to change. Other terms for neuroplasticity are brain plasticity, neural plasticity, and neuronal plasticity. The plastic nature of the human brain is evident on many levels, from molecular to behavioral.
"Neuroplasticity is defined as the ability of the nervous system to respond to extrinsic or intrinsic stimuli by a reorganization of its function, structure, or connections. It has a significant functional, but also a therapeutic, role across brain diseases, as well as in health," according to the Journal of Neuroscience.
Neurons, or nerve cells, can edit their gene expression patterns in response to dynamic environments. These changes lead to alterations in synapses, where neurons communicate with each other. When neurons fire, they release neurotransmitters from their axons into the synaptic cleft. The neurotransmitters bind to receptors on the dendrites of other neurons, which activates or inhibits their actions. The neuron that releases the neurotransmitters is the presynaptic neuron, and the one that receives the neurotransmitters is the postsynaptic neuron.
Neuroplasticity can be structural and functional. Structural plasticity refers to physical changes in the nervous system, such as brain matter volume and the number of dendrites. Functional plasticity refers to changes in the interactions between neurons, such as the strength of neural pathways.
The experiences we go through produce synaptic changes called activity-dependent plasticity. Activity-dependent plasticity, which can be functional or structural, lies at the center of neuroplasticity and is necessary for higher-level functions such as learning, memory, healing, and adaptive behavior. These changes may be acute (short-term) or long-lasting.
Why Is Neuroplasticity Important?
Without neuroplasticity, we will not be able to grow, learn, and adapt to our environments. The stories of our lives and experiences can change the structures and networks of our brains.
Neuroplasticity also plays a crucial role in adaptation to disease states and sensory deficits. Alterations in brain plasticity are associated with numerous disorders, including Alzheimer’s disease, Parkinson’s disease, anxiety, depression, post-traumatic stress disorder, and drug addiction.
To illustrate just how incredible the functional adaptability of the brain is, studies of patients with Parkinson’s disease estimate that motor symptoms do not appear until a substantial portion of substantia nigra (SN) dopamine neurons are lost. Conservative estimates set the threshold at 30% of neurons, but studies have found up to 70% neuronal loss before the onset of symptoms.
Another example comes from a study of people born blind or who became blind very early in life. The study found that reading Braille activated neurons in the visual cortex of these patients, suggesting that the neural networks had adapted to relay “tactile vision” signals. Other studies found that auditory processing in blind patients similarly activated the visual cortex.
The Science of Neuroplasticity
We must examine the cellular and subcellular levels to understand the science behind neuroplasticity. We will see how neurons alter themselves after interacting with other neurons, just as we adapt our behavior. We will first explore structural plasticity through neurogenesis before diving into the “fire together, wire together” principle of functional plasticity.
Neurogenesis
Many of us have heard that we have a set number of neurons at birth, and every neuron damaged is one crossed off the board. Although this view does reflect the relatively stable number of neurons in the adult brain, it is nonetheless outdated.
Neurogenesis is the generation of new neurons. The rate of neurogenesis is high during fetal development and early childhood but experiences a sharp drop in the late teen years and adulthood. The only adult brain structure with clearly established neurogenesis is the dentate gyrus (DG) of the hippocampus, an area of the brain critical for learning and memory.
Studies in animal and human models suggest that hippocampal neurogenesis is also involved in many cognitive and mood-related functions. These newly generated neurons may play roles in fear, anxiety, stress, pattern recognition, spatial memory, attention, etc.
While less established than the hippocampus, studies suggest that low levels of adult neurogenesis can also occur in several other areas of the brain. Specifically, adult neurogenesis may occur in the neocortex of the cerebral cortex (higher-order functions), the striatum (movement and reward pathways), and the olfactory bulb (smell processing).
Neurogenesis is crucial in maintaining cognitive abilities throughout life and adapting to some neurological conditions. However, the capacity for neurogenesis in human brains declines with age, and adult neurogenesis occurs only in specific areas of the brain. The workhorse of brain plasticity is rewiring brain circuits and not the generation of new neurons.
Fire Together, Wire Together
Canadian psychologist Donald Hebb postulated that when a presynaptic neuron repeatedly activates a postsynaptic neuron, their connection grows stronger. Other scientists nicknamed this Hebbian learning theory “fire together, wire together.” This is a great mnemonic, but we must remember that it oversimplifies the effects of timing on neural connections.
Hebbian learning forms the basis of our understanding of spike-timing-dependent plasticity (STDP), which states that the timing of stimulation between two neurons is critical in determining the outcome. If the presynaptic neuron fires just before the postsynaptic neuron, the connection is strengthened, meaning the postsynaptic neuron can now be more easily activated by presynaptic stimulation.
However, if the presynaptic neuron fires just after the postsynaptic neuron, the connection is weakened, meaning the postsynaptic neuron becomes harder to activate. If the two neurons actually “fire together” simultaneously, the strength of their connection does not change.
So far, the most accepted model of this mechanism involves a phenomenon known as long-term potentiation (LTP). In LTP, the central neurotransmitter is glutamate, the classical excitatory neurotransmitter. NMDA glutamate receptors that reside on the postsynaptic membrane mediate LTP. Magnesium ions block NMDA receptors at baseline.
The NMDA receptor expells the magnesium ion as the postsynaptic cell membrane is activated. This allows the passage of calcium ions through the NMDA receptors. The calcium ions then modify the distribution of archetypal glutamate receptors, AMPA receptors, to increase their membrane expression. Thus, the postsynaptic neurons become more sensitive to glutamate and easier to activate.
LTP works together with the related concept of long-term depression (LTD). LTD occurs when the presynaptic neuron fires too weakly to activate the postsynaptic neuron or when the postsynaptic neuron begins to fire before the presynaptic neuron.
LTD is suggested to be involved in the acute stress response and may underlie the abolition of synapses that happens in neurodegenerative diseases. For example, the pathogenesis of Alzheimer’s disease involves decreased LTP and increased LTD. However, LTP is not always good, and LTD is not always bad. Drugs like cocaine alter determinants of the LTP/LTD pathway such that their use abnormally stimulates LTP and inhibits LTD, leading to addiction.
The LTP/LTD-dependent neuroplastic pathway restructures the synapses. Synaptic plasticity underlies our ability to form memories, learn, and adapt our future behavior based on past experiences.
Neuroplasticity and Learning
The process of learning happens when an organism applies past experiences to new situations. Therefore, learning is closely related to memory formation. Researchers sought what is known as memory engrams to link brain plasticity to memory formation,
Memory engrams act as a bridge between subcellular changes to behavioral changes. Some of the most robust evidence for memory engrams came from studies on fear conditioning, which refers to an organism’s learned response to a neutral stimulus paired with an aversive stimulus.
For example, researchers played mice an auditory stimulus, say a specific tune, then administered a foot shock which caused the mice to freeze. Eventually, the mice froze in response to the auditory stimulus without the foot shock because they learned to associate the tune with pain. The study also found that the foot shock activated neurons in the amygdala, and the same neurons began to activate in response to the auditory stimulus. Therefore, a cell-level change in neural pathways explained the behavioral change. Other conditioning studies have found similar memory engrams involving the hippocampus, amygdala, and cerebral cortex.
Other researchers used optogenetic techniques to turn the process of LTP and LTD on and off in specific brain regions in mice. They found that when the optogenetic manipulation of synaptic plasticity targeted the amygdala, they could deactivate and then reactivate the neural networks for specific fear conditioning responses. In other words, they provided a direct link between synaptic plasticity and learning.
Higher-level learning processes, such as explicit memory formation, involve more complicated mechanisms. Nonetheless, synaptic plasticity, or the ability of the brain to rewire itself, adding in new connections and pruning out extraneous ones, is central to our ability to learn and grow.
Neuroplasticity and Stress
Stress is a physiological state that has broad consequences throughout the body. Under chronic stress, neurons display changed morphology. This phenomenon is evident in the hippocampus. In addition to learning and memory functions, the hippocampus interacts with the hypothalamic-pituitary-adrenal (HPA) axis, which modulates the stress response.
Under chronic stress, pyramidal cells in the hippocampus retract their dendrites. Since the postsynaptic neurons receive stimulation via their dendrites, the retraction of dendrites diminishes the efficacy of synaptic transmission and leads to reductions in hippocampal volume. Neurons in the medial prefrontal cortex show similar responses to stress. Neurons in the amygdala undergo opposite changes under chronic stress, enhancing hippocampal damage.
However, this detrimental change in neuronal morphology is reversible. As a clear display of the brain’s plastic nature, new synapses replace those lost to stress as soon as the stressor is alleviated. Drugs that aim to stimulate neuroplasticity can prevent dendritic retraction and enhance neurogenesis. Stress-induced neuroinflammation also contributes to synapse degeneration, but some anti-inflammatory drugs appear to restore neurogenesis.
Neuroplasticity and Depression
As discussed previously, neurotransmitters are molecules that act as messengers between neurons. Serotonin is an essential neurotransmitter in mood regulation. Selective serotonin reuptake inhibitors (SSRIs) are a class of antidepressants that target serotonin receptors. These drugs prevent the removal of serotonin from synapses, allowing them to stay effective for longer. Studies have shown that SSRIs reverse reductions in brain gray matter associated with depression and may increase synaptic plasticity and neurogenesis.
The serotonin-mediated enhancement of neuroplasticity is linked to a molecule called brain-derived neurotrophic factor (BDNF). BDNF is vital to neural plasticity as it regulates excitatory and inhibitory synaptic signals. Antidepressants activate the expression of BDNF, thus enhancing brain plasticity. Additionally, studies found that direct BDNF infusions to the hippocampus produce antidepressant effects, promote serotonergic neurogenesis, and increase dendritic growth.
Imaging studies in humans demonstrate that patients with depression have reduced volume in several brain structures, including the hippocampus. In addition to mood dysregulation, this can impact cognitive abilities. Antidepressants can rescue the depletion of the hippocampus, possibly through neurogenesis-dependent mechanisms. Non-medication interventions for depression, such as physical exercise, meditation, breathwork, and learning, are also shown to affect neural plasticity.
Harnessing Neuroplasticity
Although brain plasticity decreases with age, the adult brain still undergoes rewiring. Pharmacological approaches exist for some conditions affected by decreased neuroplasticity, such as antidepressants. However, there are also many non-pharmacological strategies to enhance neuroplasticity, including yoga, mindfulness training, diet, and physical exercise. These strategies generally aim to reduce stress and neuroinflammation.
Yoga, Meditation, and Breathing
As discussed previously, stress plays a major role in neuroplasticity. Mental and physical exercises that reduce stress can help harness the power of neuroplasticity. For example, various studies found that yoga, tai chi, and deep breathing exercises reduced stress and neuroinflammation markers. These exercises can buffer the effects of acute and chronic stress, reduce pain, and improve sleep quality.
Research suggests that mindfulness training and meditation can increase gray and white matter density. Additionally, general learning and enrichment can increase neurogenesis in the DG region of the hippocampus, the primary site of adult neurogenesis.
Mindfulness can rewire the brain on a structural level to produce holistic benefits. In addition, mindful training improves concentration and focus, which promotes activity-dependent brain plasticity. In other words, physical and guided mental exercises reduce stress-induced neuroinflammation and improve concentration, synergistically enhancing neuroplasticity.
Diet, Supplements, and Physical Exercise
Many natural compounds and medicinal herbs appear to have neurological benefits. One that is commonly available in supplement form is ginkgo biloba, which promotes neurogenesis and synapse formation in the hippocampus and increases the production of BDNF.
Antioxidants also have anti-inflammatory and neuroprotective effects. Antioxidants protect the nervous system from oxidative stress, damage caused by natural by-products of oxygen metabolism. The body typically produces sufficient antioxidant levels, but we can supplement this with resveratrol-containing foods, such as blueberries, cranberries, dark chocolate, and pistachios.
Exercise also supports neuroplasticity. High-intensity physical activity can induce hippocampal neurogenesis, while moderate and low-intensity activity can improve neuron survival and memory. Research suggests that physical activity also promotes hippocampal neurogenesis by increasing blood flow to the brain.
There is a caveat that high-intensity or exhaustive physical exercise can increase oxygen metabolism to the point that the body’s natural antioxidants cannot adequately counteract oxidative stress. Studies have shown that exercise such as marathon running can increase oxidative stress and inflammation and suppress immune function. However, supplementation with antioxidants and multivitamins before and after high-intensity exercise can prevent these drawbacks.
Conclusions
Neuroplasticity describes the potential for our central nervous system to change itself upon specific stimulation. The two major avenues for neuroplasticity are neurogenesis and activity-dependent synaptic plasticity. Neuroplasticity is crucial for learning, memory, and mood regulation. Decreased or altered neuroplasticity is involved in the pathogenesis of many neurodegenerative and neuropsychological disorders. Since neuroplasticity is sensitive to stress, physical and mental stress reduction exercises can help promote neuroplasticity and assist us in harboring a more healthy brain.
Frequently Asked Questions
What is neuroplasticity?
Neuroplasticity, or neural plasticity, is the brain’s ability to change its structure and functions to adapt to new experiences. It has roles in learning, memory formation, and recovery from neurological illnesses and injuries.
What is an example of neuroplasticity?
When we go through new experiences, we often use what we learn to adapt our future behavior. These changes are not just behavioral; the brain also changes its structure and signaling pathways. The brain’s plasticity is also why phantom limb pain occurs, as the brain adapts to the loss of nerve in an amputated limb.
What are the two main types of neuroplasticity?
Neural plasticity can be structural or functional. Structural neural plasticity is when the brain and neurons physically change. For example, new neurons grow via neurogenesis, or existing neurons grow new dendrites. Functional neural plasticity alters the brain’s neural networks to create or change functional outcomes.
What increases brain plasticity?
Nervous system plasticity can be protected and enhanced directly and through approaches that decrease stress and inflammation. Examples include yoga, learning, mindfulness practices, antioxidants, and physical exercise.
How is neuroplasticity related to the overarching field of neurobiology and the role of neurotransmitters?
Neuroplasticity highlights the brain's remarkable ability to reshape and evolve based on experiences and learning. This adaptive phenomenon is a specialized topic within the broader study of neurobiology. Furthermore, neurotransmitters, the brain's chemical couriers, are vital in facilitating the changes and adjustments that neuroplasticity encompasses.
ANAHANA PHYSICAL HEALTH RESOURCES
PHYSICAL HEALTH WIKIS
PHYSICAL HEALTH BLOGS
What is Central Nervous System
What is the Peripheral Nervous System
What is the Somatic Nervous System
What is the Autonomic Nervous System
References
https://linkinghub.elsevier.com/retrieve/pii/S0896-6273(13)00932-X
Clinical Progression in Parkinson's Disease and the Neurobiology of Axons - PMC
(PDF) Activation of the primary visual cortex by Braille reading in blind subjects
Recalibrating the Relevance of Adult Neurogenesis - ScienceDirect
NMDA Receptor-Dependent Long-Term Potentiation and Long-Term Depression (LTP/LTD)
Memory engrams: Recalling the past and imagining the future - PMC
Adult Neuroplasticity: More Than 40 Years of Research - PMC
BDNF — a key transducer of antidepressant effects - PMC
(PDF) Harnessing Neuroplasticity: Modern Approaches and Clinical Future
Improving the Potential of Neuroplasticity | Journal of Neuroscience
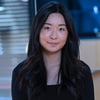
By: Emma Lee
Emma is an editor for Anahana and a soon-to-be graduate of the Master of Science program at the University of Toronto. She graduated with a Bachelor’s in Neuroscience and Immunology at the University of Toronto and has extensive experience in research. She is passionate about learning the science behind health and wellness and hopes to contribute her knowledge to help people live healthier lives. Outside of Anahana, Emma enjoys exploring nature, playing with her dog, and doing arts and crafts.